What are these striking, branching patterns, and why are they of interest to researchers? These intricate patterns, formed by electrical discharge, hold significant scientific value.
These branching patterns, often dendritic in form, arise from the non-uniform distribution of electric field strength during a discharge, typically in a dielectric material. The specific morphology of the figures, including their branching angles and fractal characteristics, reflects the material properties and the discharge conditions. For instance, variations in the dielectric's conductivity or the applied voltage will influence the shape of the resulting pattern. Visualizing these figures helps in understanding the complex interaction between electricity and matter. They are, in essence, a visual record of the electrical event.
These patterns have implications across diverse scientific fields. In materials science, they provide insights into dielectric breakdown and can be used to assess the quality and properties of insulating materials. In physics, they highlight the chaotic nature of certain electrical discharges and offer a window into non-linear phenomena. Their fascinating geometry, while seemingly chaotic, exhibits underlying structures of interest to mathematicians. Historical records showcase the fascination with these figures, dating back to early investigations into electrical phenomena, reflecting the human desire to understand the power and mystery of electricity.
Further exploration into the specific mechanisms driving the formation of these intricate structures and their implications across various fields warrants detailed study.
Lichtenberg Figures
Lichtenberg figures, captivating visual records of electrical discharges, offer insights into the intricate interplay between electricity and matter. Understanding their key aspects is crucial for appreciating their significance in various scientific domains.
- Electrical Discharge
- Dielectric Material
- Fractal Patterns
- Material Properties
- Breakdown Characteristics
- Non-linear Phenomena
- Visual Record
The intricate branching patterns of Lichtenberg figures stem from electrical discharge within a dielectric material. These fractal patterns, with their varying degrees of complexity, reflect the material's properties and the discharge's characteristics. For instance, stronger electric fields lead to more pronounced branching. The figures act as a visual record of the breakdown process, showcasing the non-linear nature of the event. Their unique structures are valuable for analyzing dielectric strength and identifying material weaknesses. The relationship between the discharge path and material properties informs analysis of insulation integrity, offering insights into material science and contributing to the understanding of various electrical phenomena.
1. Electrical Discharge
Electrical discharge is a fundamental process central to the formation of Lichtenberg figures. Understanding the characteristics of this discharge is essential for comprehending the intricate patterns that result. The discharge itself involves the movement of electrical charge through a medium, often a dielectric material. The nature of this movement and the subsequent energy dissipation are key determinants in shaping the final figure.
- Initiation and Propagation
The discharge begins with a localized breakdown in the dielectric material, creating a conductive path for current flow. This initial breakdown is often triggered by an intense electric field. Subsequent propagation depends on factors like the material's conductivity, the field strength, and the geometry of the system. This propagation process, marked by localized ionization and energy transfer, influences the branching and morphology of the final pattern.
- Non-uniformity and Field Strength
The electric field strength isn't uniform throughout the dielectric. Variations in the material's properties, such as its conductivity or composition, create localized areas of higher or lower field strength. The discharge preferentially follows paths of lower resistance and higher field strength. This non-uniformity is a critical element in the development of the intricate branching structures characteristic of Lichtenberg figures.
- Energy Dissipation and Localized Breakdown
The energy released during the discharge is not uniformly distributed. Localized breakdowns, resulting from increased field strength and ionization, lead to concentrated energy dissipation at specific points. These localized breakdowns, in turn, act as nucleation points for further branching, contributing to the complexity of the final pattern. The path of least resistance is often associated with these breakdowns, providing an avenue for further energy release and branching.
- Material Properties and Geometry
The properties of the dielectric material, such as its breakdown strength, conductivity, and structure, significantly influence the discharge's path and the resulting figure. The overall shape and geometry of the dielectric sample also play a role in shaping the discharge and ultimately, the Lichtenberg figure. Different materials respond differently to electrical breakdown, leading to varied patterns.
In summary, electrical discharge, particularly its initiation, propagation, and non-uniformity, directly impacts the characteristics of Lichtenberg figures. The discharge's intricate energy dissipation pattern, determined by material properties and geometry, ultimately creates the branching and fractal designs. This intricate relationship between electrical discharge and the resultant patterns remains a subject of ongoing study.
2. Dielectric Material
Dielectric materials are fundamental to the formation of Lichtenberg figures. These materials, characterized by their ability to store electrical energy without conducting it readily, are crucial components in the process. The intricate patterns observed in Lichtenberg figures directly reflect the inherent properties and structure of the dielectric. Variations in the dielectric's properties dictate the path of electrical discharge, leading to the distinctive branching and fractal patterns. The resulting figures become a visual representation of the material's internal structure and how it responds to the applied electrical stress.
The interplay between the dielectric and the electrical discharge is complex. The electrical field, interacting with the dielectric's internal structure, leads to localized breakdowns, or areas of higher conductivity within the material. These localized breakdowns act as focal points for subsequent discharge propagation, thus influencing the branching patterns. Differences in dielectric strength and breakdown characteristics across various materials directly affect the complexity and structure of the resulting Lichtenberg figure. For instance, a highly uniform dielectric might exhibit simpler, more radial patterns, while a material with inherent structural irregularities will likely manifest more complex, intricate branching. This understanding is crucial in assessing the suitability of materials for insulation applications, predicting potential failure points, and selecting materials for specific electrical environments. Examining these patterns provides a non-destructive evaluation technique for understanding dielectric materials' inherent weaknesses and breakdown characteristics.
In summary, dielectric materials are integral to Lichtenberg figure formation. The resulting patterns are direct visual representations of how the electrical field interacts with the material's internal structure and properties. Understanding this relationship allows for improved material selection, predictive failure analysis, and enhanced electrical insulation design. This knowledge has significant practical applications in diverse fields, from high-voltage engineering to the development of advanced materials.
3. Fractal Patterns
Lichtenberg figures exhibit fractal patterns, a recurring theme in various natural and man-made phenomena. These intricate, self-similar structures, characterized by repeated patterns at progressively smaller scales, are crucial in understanding the development and properties of these electrical discharge patterns. The presence of fractal characteristics offers significant insights into the underlying mechanisms driving the formation of Lichtenberg figures and highlights the inherent complexity of the processes involved.
- Self-Similarity and Recurrence
The fundamental characteristic of fractal patterns is self-similarity. Magnified portions of a Lichtenberg figure often resemble the overall shape, showcasing the repeating patterns across scales. This inherent recurrence suggests a process of iterative development. Each branch in the figure, in essence, has components analogous to the larger pattern, emphasizing the repeated mechanism of discharge propagation. This property is evident in various branches and sub-branches within the overall structure.
- Dimensionality and Scale-Dependence
Fractal patterns often exhibit a fractional dimension. This concept transcends the typical integer dimensions of geometric shapes, reflecting the non-uniform distribution of the electric field. The branching structures in Lichtenberg figures display this non-integer behavior, implying complex interactions at different scales. The overall dimension of the figure is often not simply a linear scaling of the smaller patterns but a more complex, scale-dependent characteristic.
- Stochastic Processes and Branching Growth
The branching patterns of Lichtenberg figures, while seemingly intricate, follow probabilistic growth patterns. This stochastic aspect is closely intertwined with fractal nature, as the path of the electrical discharge is not deterministic but involves probabilities of taking various paths. The inherent randomness in the discharge process contributes to the emergence of fractal patterns, highlighting the probabilistic nature of the physical mechanism. The occurrence of branching in diverse directions and the varied lengths of branches demonstrate this aspect.
- Implications for Material Analysis
The fractal dimension of a Lichtenberg figure can provide insights into the material properties of the dielectric. Differences in the fractal dimension between figures generated from different materials could serve as a quantitative indicator of material variations. This technique can potentially identify subtle changes or inhomogeneities in the dielectric. The fractal nature of the figure reflects the inherent characteristics of the dielectric, offering a non-destructive evaluation method.
In conclusion, the presence of fractal patterns in Lichtenberg figures is not merely a visual curiosity; it provides deeper insights into the complex interplay between electricity and dielectric materials. The self-similarity, scale-dependence, and stochastic aspects of fractal patterns highlight the iterative and probabilistic nature of the discharge process, thus providing valuable tools for analyzing material properties and understanding the underlying physics of these captivating visual representations of electrical phenomena.
4. Material Properties
Material properties exert a profound influence on the formation of Lichtenberg figures. The morphology of these figurestheir branching patterns and overall structureis inextricably linked to the inherent characteristics of the dielectric material in which the electrical discharge occurs. Variations in a material's conductivity, breakdown strength, and internal structure directly translate into discernible differences in the resulting Lichtenberg patterns. For instance, a material exhibiting high conductivity will allow the discharge to spread more readily, producing a more diffuse and less sharply defined pattern compared to a material with low conductivity, which results in a more localized, concentrated pattern.
The breakdown strength of a material, its ability to withstand an electric field without undergoing a sudden transition to a conducting state, is a critical factor. Materials with high breakdown strength generally produce figures with limited branching, suggesting resistance to electrical breakdown. Conversely, materials with lower breakdown strength will display more pronounced, extensive branching, highlighting their susceptibility to electrical discharge. Real-world applications of this understanding are found in the selection of insulating materials for high-voltage electrical equipment. Choosing materials with appropriate breakdown strength, and thus predictable Lichtenberg figures under anticipated electrical stress, is crucial for preventing equipment failure. Furthermore, by examining the patterns produced by different materials under standardized stress conditions, engineers can correlate specific Lichtenberg figure morphologies to inherent material weaknesses, aiding in the development and refinement of these crucial components.
In essence, Lichtenberg figures provide a visual representation of a material's response to electrical stress. This response, dictated by fundamental material properties like conductivity and breakdown strength, is crucial for ensuring the reliability and safety of electrical systems. By studying the intricate relationships between material properties and the resulting figures, engineers can optimize design choices, improve material selection, and ultimately enhance the performance and safety of various electrical applications. This knowledge contributes to the development of advanced materials and improves safety practices in high-voltage engineering and related fields. However, the complexity of some materials can lead to challenges in correlating specific patterns to precise material characteristics, necessitating further research to refine these interpretive techniques.
5. Breakdown Characteristics
Breakdown characteristics are central to the formation of Lichtenberg figures. These characteristics, encompassing the material's ability to withstand an electric field before exhibiting conduction, directly influence the resulting patterns. A material's breakdown strength, the maximum electric field it can endure without breakdown, fundamentally dictates the initiation and propagation of the discharge. This, in turn, determines the overall morphology of the figure. Materials with lower breakdown strength will exhibit more extensive branching, reflecting their susceptibility to electrical discharge. Conversely, materials with high breakdown strength will typically produce more localized and less extensive patterns, signifying their resilience to breakdown.
The influence of breakdown characteristics extends beyond the basic morphology. Different breakdown mechanisms, such as intrinsic breakdown, avalanche breakdown, or space charge breakdown, each contribute unique features to the Lichtenberg figure. These mechanisms reflect internal structural and compositional differences within the material. For instance, a material prone to intrinsic breakdown might show localized, highly branched patterns, indicative of its susceptibility to internal defects. The presence of impurities or voids within a material can also affect breakdown characteristics and, consequently, the patterns observed. Real-world examples include the insulation of high-voltage cables. Understanding the breakdown characteristics and resulting Lichtenberg patterns is crucial for ensuring these cables can withstand the expected electrical stress without failure. Precise characterization through these patterns allows engineers to identify and address material weaknesses.
In summary, breakdown characteristics are fundamental to interpreting Lichtenberg figures. These figures visually represent a material's response to electrical stress, with the breakdown characteristics playing a decisive role in shaping the discharge path and resulting morphology. Understanding this connection is paramount in assessing the reliability and safety of high-voltage systems, optimizing material selection for insulation purposes, and refining the process of designing electrical components. However, the complexity of some materials presents challenges in precisely correlating specific breakdown mechanisms to particular patterns, necessitating further research to refine diagnostic methodologies and improve predictive capabilities.
6. Non-linear Phenomena
Lichtenberg figures, intricate patterns resulting from electrical discharges, offer a visual representation of non-linear phenomena. The discharge's path isn't a simple, predictable response to the applied electric field, but rather a complex, often chaotic interaction. This non-linearity is evident in the branching and fractal structures that characterize the figures, making them a valuable subject for studying these complex processes.
- Nonlinearity in Discharge Initiation and Propagation
The initial breakdown in a dielectric material isn't a smooth transition but a localized, often abrupt event. The electric field's strength and the material's properties influence this breakdown, leading to a non-linear relationship between the applied voltage and the resulting discharge. This non-linearity affects where and how the discharge propagates, leading to the characteristic branching patterns. This localized breakdown acts as a trigger for subsequent branching, illustrating a non-linear feedback loop within the discharge process.
- Stochasticity in Discharge Paths
The exact path of the discharge isn't predetermined; instead, the discharge follows probabilities associated with different pathways. Variations in the material's properties, such as local variations in conductivity or impurities, create unpredictable fluctuations in the electrical field. The non-linear interplay between the field and the material properties contributes to the stochastic nature of discharge path selection, leading to the diverse and complex branching structures observed in the figures. This stochastic aspect highlights the inherent randomness of the system.
- Feedback Loops and Pattern Formation
The discharge's propagation generates localized fields and changes in the material's conductivity. These changes in turn influence subsequent discharge paths, creating feedback loops that contribute to the complex pattern development. The non-linear interactions between the electric field and the material's evolving properties lead to intricate branching patterns, with each branch creating new conditions for further discharge. The interplay of these influences illustrates the inherent non-linearity and dynamic nature of the phenomenon.
- Fractal Geometry as a Manifestation of Nonlinearity
The fractal nature of Lichtenberg figures reflects the non-linearity of the discharge process. The self-similar patterns repeated at different scales indicate a process of iterative development. The complexity arises from the non-linear interactions and feedback mechanisms. The branching and further subdivision of the discharge pathways suggest the existence of non-linear processes. This fractal geometry emerges as a direct result of non-linear dynamic interactions within the system.
In essence, Lichtenberg figures provide a visual demonstration of the interplay between non-linearity, stochasticity, and feedback mechanisms in electrical discharge. The complex and intricate patterns are not a simple response to a constant external field but a dynamically evolving system. This understanding of non-linear phenomena is crucial for comprehending the properties of materials under electrical stress, as well as the inherent complexity of certain physical processes. These figures stand as tangible representations of non-linear dynamics.
7. Visual Record
Lichtenberg figures serve as a powerful visual record of electrical discharge phenomena. Their intricate patterns encapsulate a wealth of information about the material's response to electrical stress. This visual record transcends mere aesthetics, offering a direct and often highly informative representation of the underlying physical processes. Analyzing these patterns reveals significant insights into the material's properties and the behavior of the discharge itself.
- Direct Representation of Discharge Pathways
The branching patterns in a Lichtenberg figure directly trace the paths of electrical discharge within the dielectric material. This visual representation highlights the discharge's progression, providing insights into the dominant pathways followed by the current. Differences in conductivity, breakdown strength, and material structure manifest as variations in these pathways, allowing for an almost direct visualization of the internal structure's effect on electrical flow. This is invaluable for identifying potential weaknesses or defects within the material.
- Visualization of Breakdown Mechanisms
The specific morphology of a Lichtenberg figure can often indicate the dominant breakdown mechanism at play. Localized, highly branched structures may suggest intrinsic breakdown, while more diffuse patterns might indicate avalanche breakdown. This visual distinction allows for a rapid qualitative assessment of the breakdown process. By studying the progression of the discharge and the character of the branching patterns, researchers can often infer the primary breakdown mechanisms within the material, offering a means to understand its behavior under varying electrical conditions.
- Assessment of Material Properties
Analysis of Lichtenberg figures provides a means to assess important material properties like conductivity, breakdown strength, and internal structure. The density, distribution, and overall complexity of the patterns are closely related to the material's ability to withstand electrical stress. More complex and extensive patterns often suggest lower breakdown strength or inherent structural defects, while simpler figures imply greater resistance to electrical breakdown. This visual evaluation is a non-destructive method, providing valuable information about material quality without requiring a material sample to be destroyed or altered.
- Historical Context and Scientific Advancement
The recognition of Lichtenberg figures as a visual record of electrical discharge has contributed significantly to scientific understanding over time. Early experiments and observations of these figures laid the groundwork for understanding electrical phenomena. Their study has led to further investigation into dielectric breakdown and material behavior under electrical stress, advancing scientific understanding across various disciplines. The historical context underscores their enduring value as a visual record of significant scientific discoveries.
In conclusion, the visual record offered by Lichtenberg figures transcends their aesthetic appeal. The intricate patterns meticulously capture the discharge's path, breakdown mechanisms, and material properties. This visual approach provides researchers with a valuable tool for understanding and evaluating dielectric materials, contributing to advancements in various scientific and engineering fields. The historical significance further emphasizes the importance of this visual representation in the evolution of scientific knowledge regarding electrical discharges and material behavior.
Frequently Asked Questions about Lichtenberg Figures
This section addresses common inquiries regarding Lichtenberg figures, aiming to provide concise and informative answers. These figures, resulting from electrical discharges, offer valuable insights into material properties and electrical phenomena. Understanding these patterns is crucial across various scientific disciplines.
Question 1: What are Lichtenberg figures, exactly?
Lichtenberg figures are intricate, branching patterns formed by electrical discharges within a dielectric material. The patterns' morphology reveals details about the material's electrical breakdown characteristics, including conductivity, strength, and internal structure. These patterns are not random but reflect the interplay between the electric field and the dielectric's inherent properties.
Question 2: How are Lichtenberg figures formed?
The formation involves an electrical discharge within a dielectric material. This discharge, initiated by a localized breakdown, propagates along paths of least resistance. Variations in the material's properties lead to non-uniform electric fields, resulting in the characteristic branching patterns. Factors such as the electric field strength, material conductivity, and geometry of the sample influence the final figure.
Question 3: What do these figures tell us about the material?
The morphology of a Lichtenberg figure provides information about a material's breakdown characteristics. The extent of branching, the overall pattern complexity, and the presence of localized areas reveal insights into the material's conductivity, strength, and internal structure. Analysis of these visual patterns can identify weaknesses and potential failure points, especially in high-voltage applications.
Question 4: Are Lichtenberg figures only found in laboratories?
While laboratory demonstrations are common, Lichtenberg figures can be found in various contexts where electrical discharges occur. Examples include lightning strikes on certain surfaces, instances of electrical equipment failure, or even in specific types of experimental settings. In each case, the patterns reflect the interplay between electricity and the material involved.
Question 5: What are the practical applications of understanding Lichtenberg figures?
Understanding Lichtenberg figures assists in material selection for high-voltage applications, allowing engineers to choose materials with appropriate breakdown characteristics. Analysis of these figures also facilitates a non-destructive evaluation of existing materials, identifying weaknesses and potential failure points. The knowledge gained is critical in preventing electrical equipment failures and ensuring system safety.
In summary, Lichtenberg figures are more than just captivating visuals; they represent a window into the complex interactions between electricity and matter. Understanding these figures provides valuable insight into material properties, enabling informed decisions in various scientific and engineering applications.
Further exploration into specific applications and the relationship between different material properties and corresponding figure morphologies is a subject worthy of detailed study.
Conclusion
Lichtenberg figures, intricate patterns arising from electrical discharges in dielectric materials, offer a compelling visual representation of complex physical processes. This exploration has highlighted the intricate relationship between the morphology of these figures and the underlying material properties. Key aspects examined include the interplay between electrical discharge paths and material conductivity, the manifestation of non-linear phenomena in the discharge propagation, and the fractal geometry embedded within the patterns. The analysis underscored how breakdown characteristics, such as a material's resistance to electrical stress, are directly reflected in the figure's complexity and branching structure. Lichtenberg figures thus provide a valuable non-destructive method for assessing material properties relevant to electrical insulation and high-voltage applications.
The study of Lichtenberg figures extends beyond a purely visual observation. Understanding the underlying physics of their formation provides insights crucial for optimizing material selection in high-voltage engineering, improving the reliability of electrical systems, and enhancing safety protocols. Future research could focus on refining diagnostic methodologies, correlating specific figure morphologies to precise material characteristics, and exploring the application of Lichtenberg figure analysis in emerging technologies, such as advanced dielectric materials. This detailed understanding of electrical discharges and material behavior holds significant implications for the development of safer, more efficient electrical infrastructure and technological advancements.
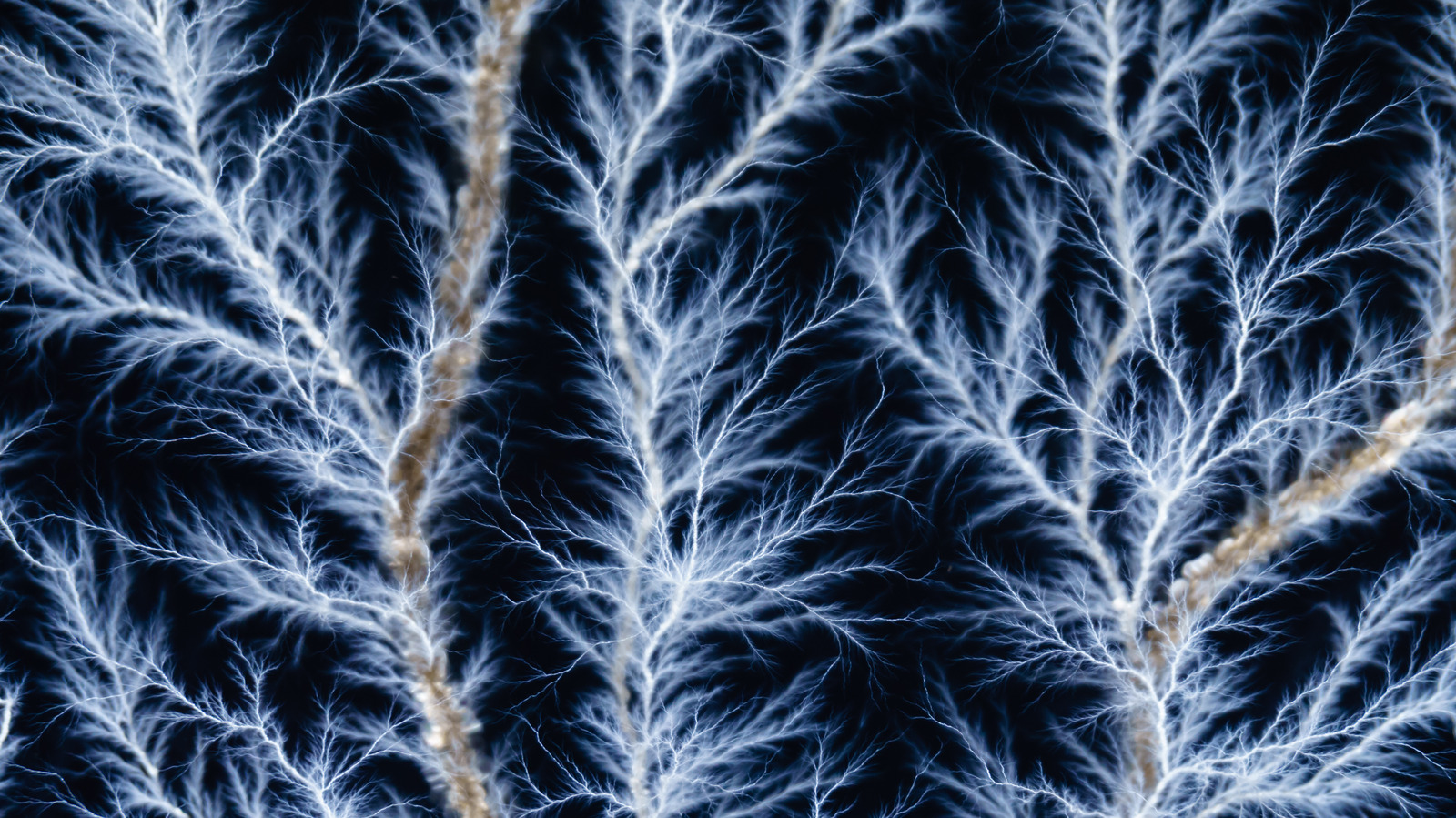
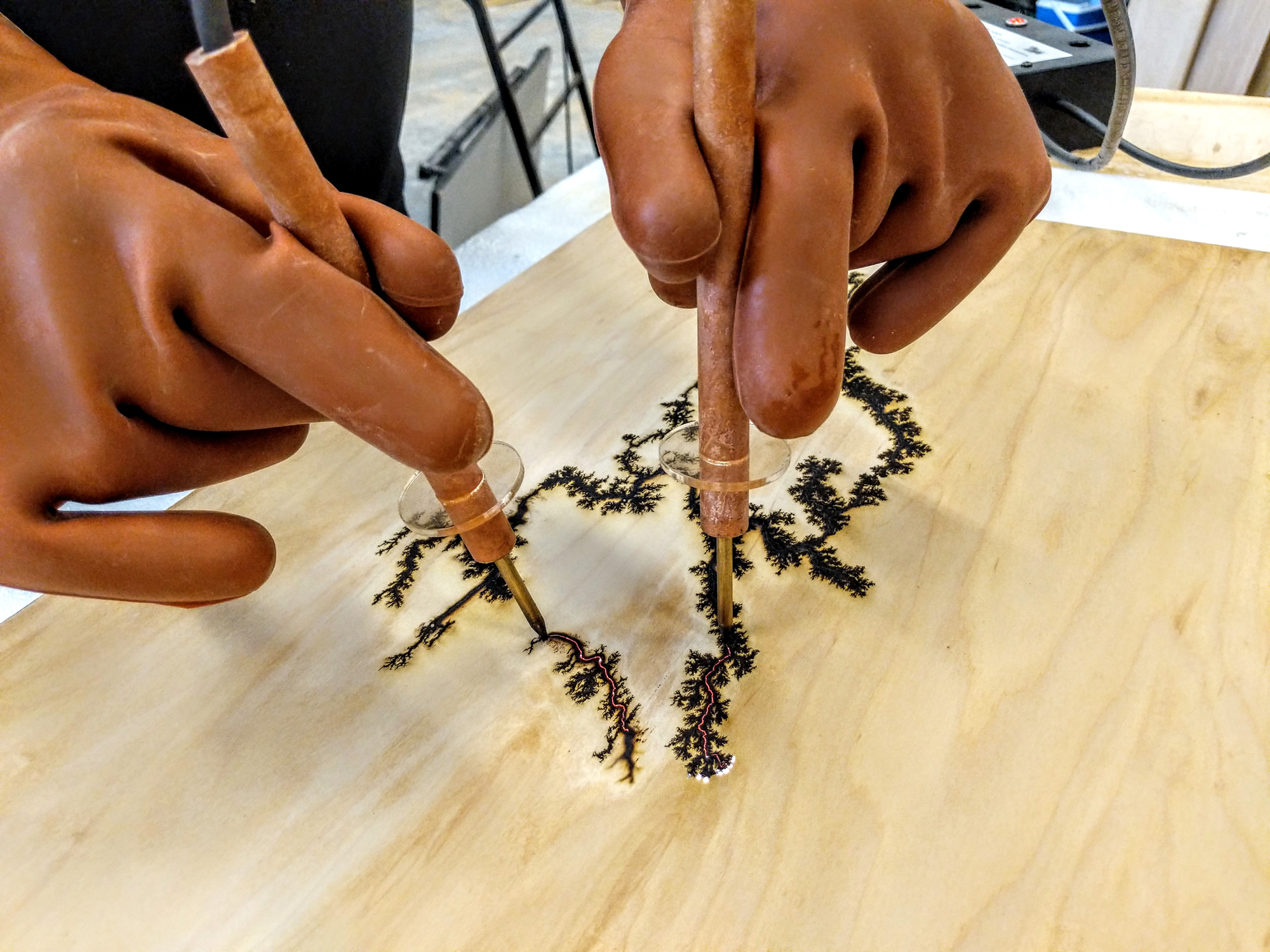
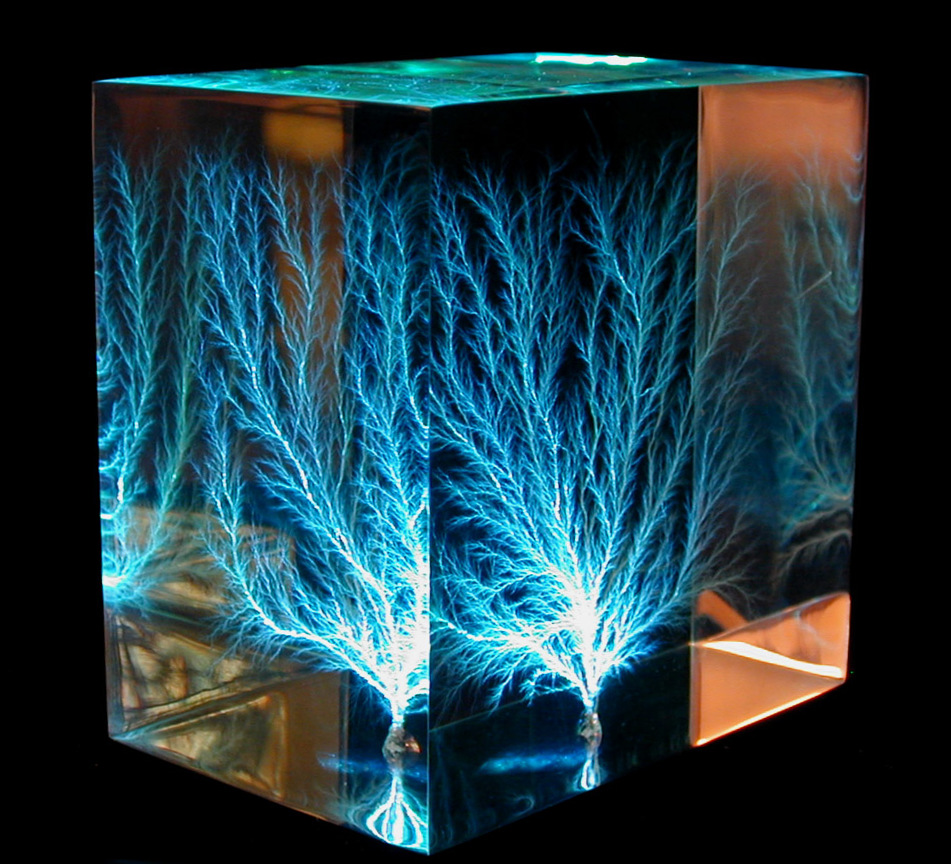